3d Resistivity Inversion Software Downloads
ERTLab Solver is the resistivity and IP inversion module that offers full three-dimensional topographical modelling and inversion. Its numerical core based on tetrahedral FEM allows inputting of complex topographic information and inverting data sets collected using surface, borehole and surface-to-hole array configurations.
Most popular related searches
ERTLab Sequencer is a practical tool for the creat...
ERTLab Sequencer is a practical tool for the creation of 2D and 3D arrays/schedules of electrical resistivity measurements.
ERTLab Viewer is a powerful graphical environment ...
Calculate plane wave resistivity and phase. 3-D and 2-D model presentation. Inversion of 3-D model. 3-D MT modeling and inversion. KMS Technologies – KJT Enterprises Inc. And their alliance partners have developed a software packages to conduct 3-D land/marine MT modeling and inversion for oil companies.
ERTLab Viewer is a powerful graphical environment to represent resistivity/IP models. Visualize inverse and forward models in a variety of ways, including sections, volumes and isosurfaces. These may be spun and re-oriented in real-time.
Downloads related to this software
Request a demo
Abstract
The resistivity method is frequently used in the investigation of the shallow parts of the earth. Interpretation of such data is usually done assuming a layered earth. However, a more complete imaging can be obtained if 3D models are used. Thirty-five vertical electrical soundings (VES) were carried out in a regular mesh at the northwestern part of Greater Cairo in order to characterize different geological units and to study their quality for building foundations. Models obtained from 1D inversion of each VES, together with borehole information, were used for construction of eight geoelectrical sections which exhibit the main geoelectrical characteristics of the geological units present in the area. The 3D inversion of the data indicated a complex subsurface electrical resistivity distribution conditioned by lithology, water content and tectonic structures. The results indicate that the subsurface consists of different geologic units such as gravel and sand, sand, clay and limestone. The main results are related to the characterization of the clay formations in the north of the survey area, which is revealed by low-resistivity values (<100 Ω m) and sand layers associated with high-resistivity values (>600 Ω m) depicted in the central part of the study zone.
1 Introduction
The construction of any structure in civil engineering demands the knowledge of soil engineering properties obtained by geotechnical tests. However, the number of test sites is limited, demanding extrapolation/interpolation of the results over a larger area. The use of geophysical methods in civil and environmental engineering has increased in the past few years helping to produce accurate extrapolation/interpolation of soil geotechnical properties (Ward 1990, Dannoski and Yaramanci 1999, Cosenza et al2006, Giao et al2003, Sultan et al2004, Soupios et al2007). Resistivity methods are widely used in ground investigation for building foundation analyses, inspection and monitoring of dams and dikes (Karastathis et al2002, Abu-Zeid 1994) and detection of potentially dangerous structures in the subsurface (Debeglia and Dupont 2002).
Resistivity data are usually interpreted assuming one-dimensional (1D) or two-dimensional (2D) models (Koefoed 1960, Loke 1997). The use of three-dimensional (3D) modelling and inversion of resistivity data has increased in the past few years taking advantage of the great development on the automatic data acquisition systems and faster computers and mathematical algorithms. Several algorithms have been developed to perform 3D resistivity modelling and inversion. 3D forward algorithms based on the finite-difference, finite-element and integral methods have been presented by several authors (Spitzer 1998, Zhang et al1995, Zhao and Yedlin 1996, Loke and Barker 1996, Tsourlos and Ogilvy 1999, Yi et al 2001, Pidlisecky et al2007, which are just some of the papers published in the last 10 years).
Several authors have published examples of the application of 3D modelling and inversion of resistivity data. Monteiro Santos et al (1997) used a 3D approach in modelling resistivity rectangle and dipole–dipole data. Park (1998) applied the method to monitor the movement of a fresh water plume through a vadose zone. Ogilvy et al (1999) applied the 3D inversion to investigate industrial waste deposits. Weller et al (2000) applied 3D inversion in order to better characterize the induced polarization data of a simulated waste site. Stoll et al (2000) reported the 3D modelling of double-dipole data. Represas et al (2005) used 3D inversion of resistivity data for geological and mining investigation. El-Quady et al (2005) and Sultan et al (2006) applied 3D inversion of resistivity data investigating archaeological sites in Egypt. Recently, Soupios et al (2007) have used 2D and 3D inversion of dipole–dipole data in combination with geological and geotechnical data to investigate a vacant building site.
In this study, the authors used 1D and 3D interpretation of resistivity data (Schlumberger soundings) to characterize the different geological units that represent the stratigraphic section of the study area and to study their quality for building foundations.
The study area stands at the northwestern part of Greater Cairo, northward of the Cairo–Suez road, and is located between latitudes of 30° 06′ 30″ and 30° 08′ 30″ E and longitudes of 31° 39′ 00″ and 31° 41′ 30″ N (figure 1). The study area represents a flat area between El Shourk and Bdr cities and was intended to be prepared for different types of constructions.
Location and geological map of the study area (modified after the internal report of the Geological Survey of Egypt).
Location and geological map of the study area (modified after the internal report of the Geological Survey of Egypt).
2 Geology of the study area
The geology of the area was studied by the geological survey of Egypt (EGSMA 1996). The surface geology consists of different units with ages of Quaternary, Middle Miocene and Oligocene (figure 1). The Quaternary deposits occur in the eastern and southeastern parts of the study area and also cover the wadies that cross the studied area. The Quaternary deposits are composed of sand dunes, sand sheet and alluvium deposits. The Middle Miocene deposits occur in the northern part of the area and are represented by Hommath formation, which consists of sandy limestone, clay and calcareous sandstone. Oligocene deposits represented by Gabal Ahamar formation composed of sandstone, loose sand and gravel cover the central part of the study area. Three boreholes, drilled in the study area by the geological survey of Egypt (EGSMA 1996), with depths of 50 m indicate that the shallow part of the subsurface section consists of different varieties of sand, sandstone, clay and limestone (figure 2).
Wells geological logs. 1: Gravel and sand, 2: sandstone, 3: coarse and fine sand, 4: coarse sand, 5: silty sand and 6: fine and medium sand.
Wells geological logs. 1: Gravel and sand, 2: sandstone, 3: coarse and fine sand, 4: coarse sand, 5: silty sand and 6: fine and medium sand.
3 Geoelectrical data and interpretation
Thirty-five vertical electrical soundings (VES) were carried out in the area under investigation (figure 3) in a regular grid with spacing between soundings of approximately 500 m. The VES were executed using a Russian electronic compensator, type AE-72. The maximum current electrode spacing (AB) was chosen to be 500 m, aiming to obtain information about the thicknesses and resistivities of the shallow subsurface section. The VES field apparent resistivity curves are shown in figure 4. Three/four-layer models can in general explain the main features of the curves. There are curves of the types H, A and Q. The H-type curves, revealing a second conductive layer, are dominant in the northwestern and southeastern parts of the survey. The A- and Q-type curves are mostly represented in a SW–NE region that crosses the central part of the survey area. The pattern of the curves changes slowly between sites. Exceptions are, however, observed in the vicinity of the lithological changes which are, in general, associated with tectonic structures like faults. Example of variations greater than one decade are observed between VES 11 and 6 in the northwestern part and between VES 31 and 32 in south of the area, both associated with probable faults crossing the area.
Location map for vertical electrical soundings (VES) and boreholes.
Location map for vertical electrical soundings (VES) and boreholes.
VES data (symbols) and model responses (lines) of the 3D model shown in figure 8, for selected sites.
VES data (symbols) and model responses (lines) of the 3D model shown in figure 8, for selected sites.
3.1 1D resistivity interpretation
VES apparent resistivity data were inverted assuming layered-earth models to determine the thicknesses and true resistivities of the successive strata below each VES site. There are several interpretation methods; some of them are graphical (manual) and others are numerical methods. The authors used manual interpretation based on two-layer standard curve Cagniard graphs (Koefoed 1960) to estimate initial models for the inversion process using the IPI2WIN 2000 software. The IPI2WIN program deals with VES curves in the man–computer interactive regime and draws theoretical and field curves on a display screen together with the Rho(z) model curve.
Three boreholes drilled in the area, where three VES were measured, were used for the correlation and calibration between the interpreted electrical data and the real geologic information. Figure 5 shows a comparison between the resistivity models and the geological logs. VES 13 and borehole D5 are located in the Gabal Ahamar formation dominated by fine and medium sand. The variation on the resistivity values is conditioned by the presence of the groundwater in the medium grain size material (corresponding to the 100 Ω m layer at depths between 8 and 20 m). At depths between 20 and 80 m, the high-resistivity value (2000 Ω m) is probably justified by the presence of fine sand, which is less porous and dry. The new decrease in resistivity detected at 80 m depth cannot be correlated with any borehole information, but it is probably due to the presence of sandstone. Sounding VES 31 and borehole F1 were performed in Quaternary sedimentary formations. A quite good correlation between resistivity and geological layers can be noted in the upper part of well F1 and VES 31. The relative low-resistivity layer (90 Ω m) starting at a depth of approximately 32 m, where the sandstone layer starts, might represent the aquifer.
Comparison between 1D models obtained from inversion of VES 31 and 13 with geological logs from wells F1 and D5, respectively.
Comparison between 1D models obtained from inversion of VES 31 and 13 with geological logs from wells F1 and D5, respectively.
The results of 1D inversions were combined with the geological information from boreholes in the construction of eight interpreted cross-sections: seven of them are in the W–E direction and the eighth is in the S–N direction (figures 6 and 7). Geoelectrical cross-sections along profiles A-A′ and B-B′ cross the northern part of the survey area represented by wadi deposits of Quaternary age and by the Middle Miocene deposits (Hommath formation composed of sand and gravel, clay and calcareous sandstone). The clay layer of Middle Miocene exhibits low-resistivity values ranging from 2 to 10 Ω m. The models suggested the presence of this clay layer in the whole north part of the survey area. This layer acts as the north limit of the aquifer.
W–E geoelectrical cross-section with results of boreholes, resistivity values in Ω m.
W–E geoelectrical cross-section with results of boreholes, resistivity values in Ω m.
Geoelectric cross-section along profile H-H′, resistivity values in Ω m.
Geoelectric cross-section along profile H-H′, resistivity values in Ω m.
The geoelectrical cross-section along profile C-C′ represents formations of three ages: Quaternary (wadi deposits), Middle Miocene (calcareous sandstone) and Oligocene deposits (sand and sandstone). This last layer of sand contains the groundwater aquifer and exhibits resistivity ranging from 72 to 152 Ω m but the dry sand reveals very high-resistivity values ranging from 297 to 1810 Ω m. There are variations in the thicknesses of the different types of sand according to grain size. These variations are a consequence of the deposition cycles. The central part of the study area includes the geoelectric cross-section along profiles D-D′, E-E′ and F-F′ that exhibit the wadi deposits overlaying the Oligocene sand. The geoelectric cross-section along profile G-G′ crosses the southern part of the area, where the western part is represented by Oligocene deposits and the eastern part is represented by wadi deposits of Quaternary age with the thickness of about 40 m overlaying the Oligocene deposits. A normal fault (F1) dissecting the surface and subsurface stratigraphic units intercepts the profile between VES 31 and 32 with the downthrown towards VES 32. The geoelectric cross-section H-H′ (figure 7) crosses the area in the N–S direction including VES 31, 26, 16, 11, 6 and 1. This section crosses the Quaternary, Middle Miocene and Oligocene deposits. A probable normal fault is located between VES 6 and 11 where the contact between Middle Miocene and Oligocene deposits is mapped.
The 1D geoelectrical cross-sections suggest that the subsurface structure is more complex than initially suspected. Therefore, a 3D model is more adequate for its interpretation.
3.2 3D resistivity interpretation
The 3D inversion code used in this work was developed using the finite-element approach for forward calculations and a smoothness-constrained least-squares algorithm for inversion (Monteiro Santos et al1997, Represas et al2005). The program uses an irregular mesh constructed for each VES site, taking into account sounding parameters (AB/2 spacing and spatial orientation). With the meshes adopted in this work a numerical error lower than 3% is expected in the apparent resistivity calculations.
The 35 VES, corresponding to 490 data measurement points, were inverted considering the subsurface domain divided into 1920 hexahedral elements of unknown resistivity. Horizontally, the finite-element mesh was defined for each sounding, in general, with 91 × 96 nodes. In the downward direction the mesh spacing increases according to the exponential law Δz = Δzmexp(0.2 nz), where Δzm represents the thickness of the uppermost hexahedral element (0.5 m in this case) and nz is the vertical level of the element. Twenty-eight nodes were used in the vertical direction. A 230 Ω m (corresponding to the average apparent resistivity data) uniform medium was considered for an initial model. The regularization algorithm proposed by Sasaki (1989) was adopted in this work. The results presented in this section have been obtained using a regularization parameter of 0.3, which corresponds to the better fitting between data and model responses. This result was obtained after several inversion runs with different parameters. An initial homogeneous medium of 230 Ω m was used. This value corresponds to the average of the measured apparent resistivity values.
The results are presented in figure 8 as horizontal slices of the resistivity model. This model was obtained after 16 iterations. The comparison between measured and calculated apparent resistivity curves is shown in figure 4. The misfit between data and model responses varies between soundings: a huge misfit is observed at site 33 (VES 33). A high misfit is observed at shortest AB/2 spacing for VES 11, 12, 17, 25 and 28. For the majority of the soundings the fit is quite good, with rms error lower than 12%.
Horizontal slices from the 3D resistivity model. Dashed lines in the first slice represent the contours of the main geological units. VES location is represented by crosses. Tm: Miocene deposits, Q: Quaternary deposits and To: Oligocene deposits.
Horizontal slices from the 3D resistivity model. Dashed lines in the first slice represent the contours of the main geological units. VES location is represented by crosses. Tm: Miocene deposits, Q: Quaternary deposits and To: Oligocene deposits.
The Hommath formation (sandy limestone, clay) at the northern part of the area is revealed as a low-resistivity zone (resistivity < 100 Ω m). The high content in clay might be the reason for the low-resistivity values. This agrees with the results obtained from 1D inversion for the intermediate layers. The main differences between 1D and 3D models in this part of the survey area are noted in the deep structures that were revealed to be more conductive in the 3D model—resistivity values lesser than 100 Ω m are noted in the 3D model in contrast to the 1D results, where values ranging from 150 to 200 Ω m are observed. The central part of the survey area, which corresponds to the Gabal Ahmar formation, is characterized by high-resistivity values (>500 Ω m) alternated with low-resistivity values. This discontinuous pattern can be an artefact originated by the large spacing between VES sites. The high-resistivity values become more continuous and dominant with increasing depth. These high-resistivity values might correspond predominantly to gravel and loose sand. The low-resistivity values might be due to the groundwater presence. The transition between high- and low-resistivity zones in the southern and eastern parts of the survey area correlates very well with the transition from Gabal Ahmar and Quaternary formations mainly represented by alluvium, sand dune and sand sheet. The presence of groundwater might be responsible for the low resistivity of these formations. In the south the transition between Gabal Ahmar and Quaternary formations is represented by a normal fault. To the east the 3D resistivity model suggests that the Gabal Ahmar formation is deeper and covered by Quaternary sediments. The 3D model agrees, in general, with the 1D ones. The main difference is verified in the northern part of the survey area for deep structures. This difference will be analysed in the next section.
4 Sensitivity tests
The sensitivity of the data to some features in the 3D model was studied considering two models derived from the final inversion model shown in figure 8. In the first model (A) the resistivity of all the elements between 300 and 700 Ω m was changed to 300 Ω m. This change mainly affects the structures in the central part of the survey area. For the soundings located to the north and south of the survey, the changes on apparent resistivity curves correspond to changes on lateral structures. In the second model (B) the resistivity of all the elements at a depth greater than 100 m was changed to 700 Ω m. This change corresponds to an increase in the resistivity of deep northern and southern structures. This also corresponds to a slight decrease of the deep structures in the central part of the survey area.
Model A allows us to study the importance of the high resistivity (mainly associated with the Oligocene formations in the centre of the survey area) in the model responses. Model B was motivated by the discrepancy observed in deep structures in 1D and 3D models and the misfit between data and the 3D model response for largest AB/2 spacing at some soundings located in the north part of the area (e.g. in VES 3). In contrast to the 3D model, the 1D models obtained from VES located in the northern part of the survey area suggest resistive deep bedrock (probably made up of limestone).
The model responses at nine selected sites are shown in figure 9. In this figure the responses obtained with models A and B are compared with experimental data and with the responses of the best-inverted model shown in figure 8.
Results of the sensitivity tests at some chosen sites. Dots are the observed data, best 3D resistivity model responses are represented by a continuous black line (1), responses for the model where resistivities in the range 300–700 Ω m are changed to 300 Ω m are represented by a dashed-dot line (2). The short-dashed line represents the responses of a model with structures deeper than 100 m changed to 700 Ω m (3).
Results of the sensitivity tests at some chosen sites. Dots are the observed data, best 3D resistivity model responses are represented by a continuous black line (1), responses for the model where resistivities in the range 300–700 Ω m are changed to 300 Ω m are represented by a dashed-dot line (2). The short-dashed line represents the responses of a model with structures deeper than 100 m changed to 700 Ω m (3).
The decrease in the resistivity (model A) does not affect soundings located to the north and south out of the survey area (VES 3, 8 and 33), showing that high apparent resistivity measured for large AB/2 spacing is not originated by lateral effects caused by the high-resistivity central structures. Soundings carried out in the Oligocene formations (VES 13, 18, 23 and 28) have great sensitivity to this decrease in resistivity suggesting that high-resistivity Oligocene formations are thick.
Soundings located in the northern part of the area, such as VES 3, show a better fit (mainly for large AB/2 spacing) when the resistivity of deep structures increases (model B). This shows that deep structures in that part of the 3D model are not well resolved. Soundings carried out in the centre of the survey area (VES 18, 23) are not affected by resistivity changes originating from model B. Soundings located in the Quaternary formations (VES 16, 28 and 33) have strong sensitivity to deep resistivity, showing that deep structures in the southern part have resistivity significantly lesser than 700 Ω m.
5 Discussion
Attempts to relate geotechnical properties (such as unit weight, water content, liquid limit, plastic limit, liquidity index, undrained shear strength, compression ratio, effective stress, grain size dimension, cone resistance, among several others) to geoelectrical results are not so frequent. The relatively few published results relating mechanical properties to electrical properties seem to be contradictory (Cosenza et al2006, Endres and Clement 1998, Braga et al1999, Giao et al2003). The only correlation that seems to be well understood and well established is the interdependence between electrical resistivity and water content.
A relatively good correlation between the 1D and 3D resistivity models and the geology can be noted for the upper layers. The relatively high resistivity (160 Ω m) at depths between 18 and 40 m represents the combined response of the gravel and of the coarse and fine sand formations. In the north part of the survey area the models show a decrease in the resistivity (to 2–5 Ω m in 1D models) approximately at 10–20 m depth. This change in the resistivity correlates quite well with the beginning of a clay layer.
Clay layers are important in civil engineering. For example, clay minerals have a great affinity for water. Some clay minerals swell easily and may double in thickness when wet. The process by which some clay minerals swell when they take up water is reversible. Swelling clay expands or contracts in response to changes in environmental factors (wet and dry conditions, temperature). According to Velde (1995), hydration and dehydration can vary the thickness of a single clay particle by almost 100%. Therefore, any construction built on soil containing swelling clays may be subject to structural damage caused by seasonal swelling of the clay portion of the soil.
6 Conclusions
Geophysical surveys can contribute to identify subsurface zones which are inappropriate for the construction of building foundations. Electric imaging produced from resistivity data proved to be a useful tool in clay layer detection.
The results presented here show that the survey area has a complex subsurface electrical resistivity distribution, conditioned by lithology, water content and tectonic structures. From a civil engineering point of view the main results are related to the characterization of the clay and sand (Oligocene age) layers. Clay soils are more susceptible to subsidence being also strongly affected by environmental conditions (Soupios et al2007). Therefore, special care is recommended if foundation footings are to be constructed in the northern part of the survey area.
Acknowledgments
The first author (Sultan Awad Sultan) is indebted to the Fundação para a Ciência e Tecnologia (Portugal) for his support through the post-doctor fellowship of reference (SFRHBPD221162005). This work was partly developed in the scope of the scientific cooperation agreement between Centro de Geofisica da Universidade de Lisboa, Portugal (CGUL) and the National Research Institute for Astronomy and Geophysics, Egypt (NRIAG).
References
Journal Of Geophysics And Engineering
)http://dx.doi.org/10.1007/s1004000500551431217414350157Fernando A Monteiro Santos
. ,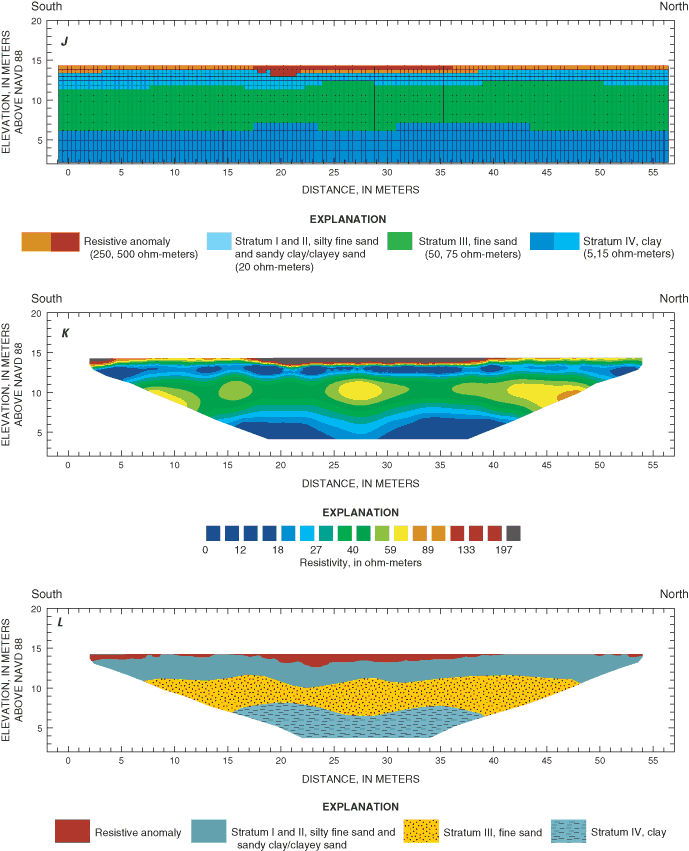
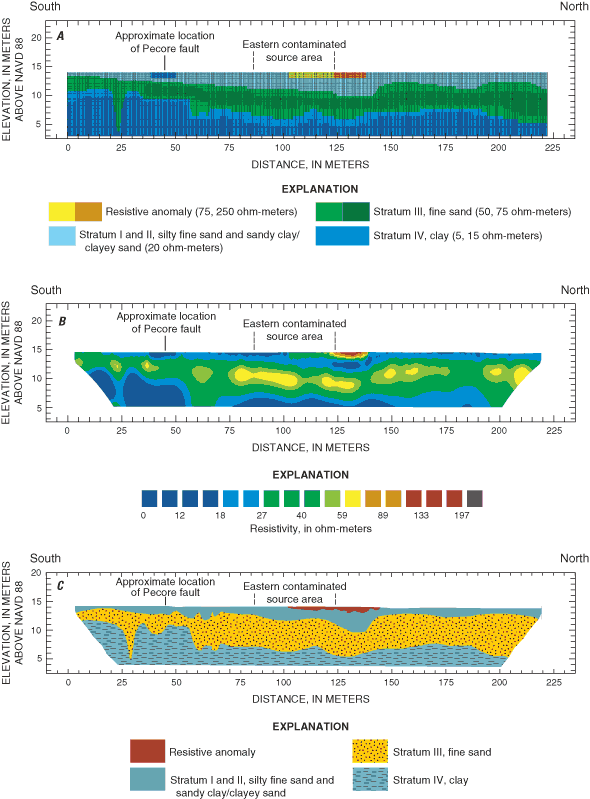
